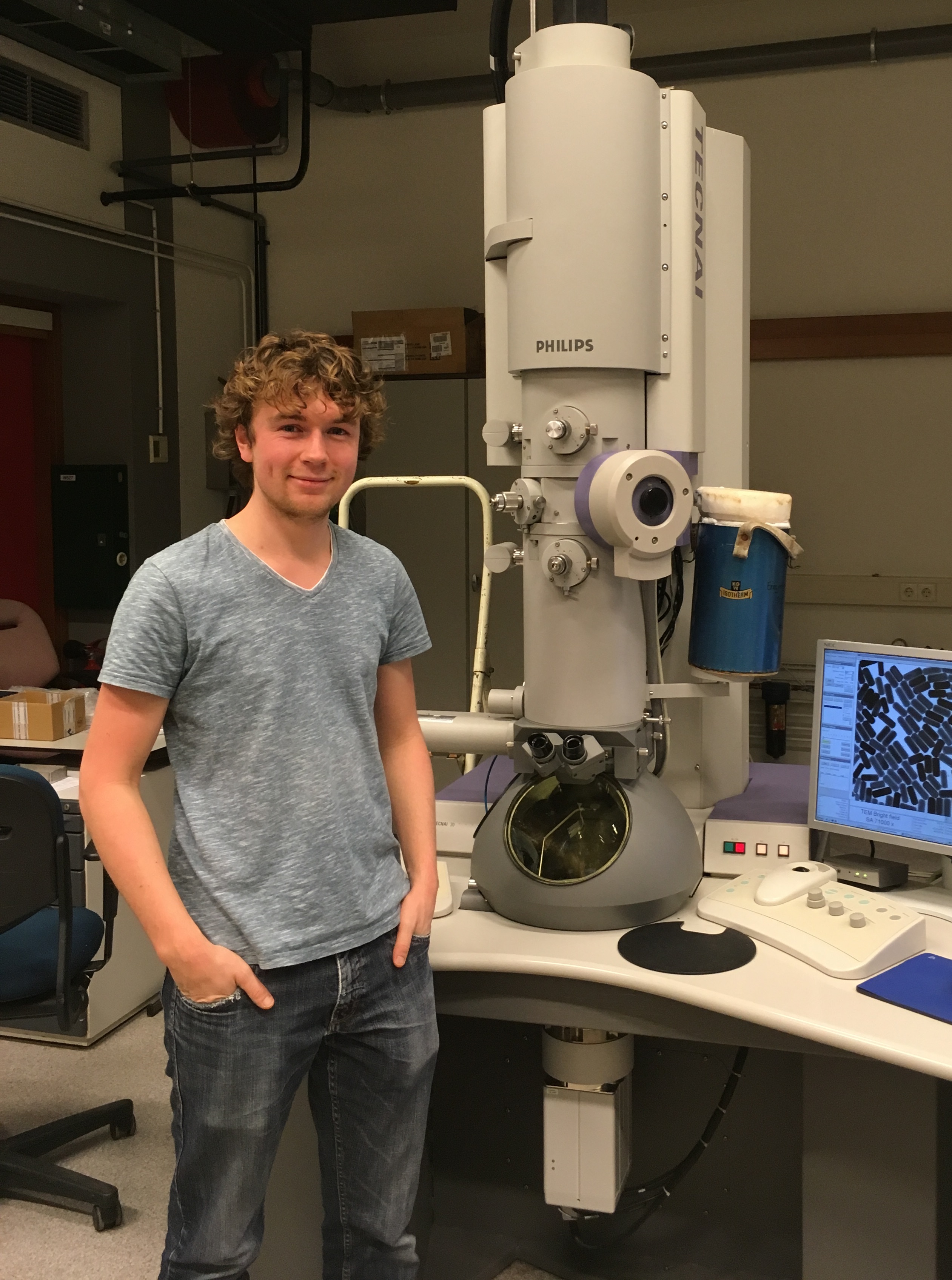
Maarten Bransen MSc
Leonard S. Ornstein Laboratory, room 0.18
Princetonplein 1, 3584 CC Utrecht
P.O. Box 80 000, 3508 TA Utrecht
The Netherlands
phone: +31 (0)30 253 2315
secretariat: +31 (0)30 253 2952
e-mail: m.bransen@uu.nl
Research
Promotor: Prof. dr. Alfons van Blaaderen
Funding: NWO/MCEC
Employed: 1 December 2017 – 30 November 2021
Measuring nanoparticle interactions
Colloidal nanoparticles can spontaneously arrange themselves into well-defined structures in a process called self-assembly (SA). Such self-assembled structures can have unique optical, structural and electronical properties due to the nanoscale nature of the individual components as well as the local or long range order in the system. Self-assembled materials of nanoparticles may be used for a wide range of applications, such as (but not limited to) photonic circuits, (photo)catalysis, nanosensing, drug-delivery, photovoltaics and data storage [1,2].
The mechanisms by which SA processes occur are governed by the interactions between the nanoparticles. These interactions can be a combination of many effects such as van der Waals attraction, excluded volume effects, screened Coulomb repulsion and steric repulsion of ligands. Theoretical models for colloidal interactions (e.g. DLVO theory) however are no longer accurate when the particle size is in the nanoscale regime (~1 to 100 nm) because assumptions like a continuum treatment of the solvent and additivity of interactions break down. Better understanding and experimental measurement of these interactions is thus of high interest for studying and predicting the SA process and the resulting structures [3].
The aim of my project is to measure the interactions between different nanoparticles in colloidal suspension in real space and in 3D using optical and electron microscopy. From measurement of the particle positions, the radial distribution function g(r) can be obtained which describes the probability of different particle-particle distances r. From the g(r) the pair interaction potential U(r) can be directly obtained, which was achieved for the first time in our group for micron-sized colloids using 3D scanning confocal microscopy [4]. So far this has not been possible for nanoparticles because of the fast dynamics and the high resolution required.
Using cryogenic freezing or a novel UV-initiated polymerization of the solvent, the particles can be arrested on a very short timescale, which allows for determination of their positions with either high resolution scanning confocal microscopy, focused ion beam milling in combination with scanning electron microscopy (FIB-SEM) or transmission electron tomography (TEM-tomography). In addition to measuring the g(r), the particle interaction potentials may be obtained from following particle trajectories over time [5]. Using our new ultrafast confocal microscope it may be possible to follow nanoparticle dynamics in real-time and obtain the interaction potential in this way.
[1] Nie, Z., Petukhova, A., & Kumacheva, E. (2010). Nature Nanotechnology, 5(1), 15–25. https://doi.org/10.1038/nnano.2009.453
[2] Boles, M. A., Engel, M., & Talapin, D. V. (2016). Chemical Reviews, 116(18), 11220–11289. https://doi.org/10.1021/acs.chemrev.6b00196
[3] Silvera Batista, C. A., Larson, R. G., & Kotov, N. A. (2015). Science, 350(6257), 124277. https://doi.org/10.1126/science.1242477
[4] Royall, C. P., Leunissen, M. E., & Van Blaaderen, A. (2003). Journal of Physics: Condensed Matter, 15(48), 3581–3596. https://doi.org/10.1088/0953-8984/15/48/017
[5] Jenkins, I. C., Crocker, J. C., & Sinno, T. (2015). Soft Matter, 11(35), 6948–6956. https://doi.org/10.1039/C5SM01233C
This work is supported by the Netherlands Center for Multiscale Catalytic Energy Conversion (MCEC), an NWO Gravitation programme funded by the Ministry of Education, Culture and Science of the government of the Netherlands.