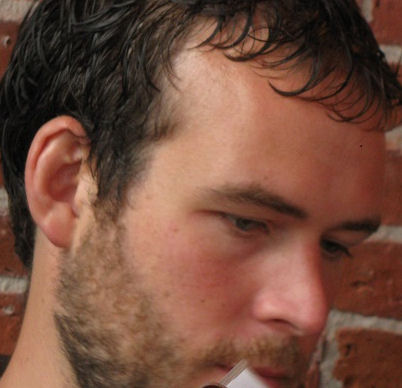
Wessel Vlug
Ornstein Laboratory, room 0.12
Princetonplein 1, 3584 CC Utrecht
P.O. Box 80 000, 3508 TA Utrecht
The Netherlands
phone: +31(0)30 253 2925
secretariat: +31(0)30 253 2952
e-mail: w.s.vlug@uu.nl
Research
Supervisor: Dr.ir. M.A. van Huis
Promotor: Prof.dr. A. van Blaaderen
Employed since March 2012
Funded by FOM
Colloidal synthesis, Confocal laser scanning microscopy (CSLM), Particle tracking, Transmission electron microscopy (TEM)
The colloidal domain extends from roughly 10 nm to 1 μm. Colloidal particles are excellent model systems as they are large and slow enough to be observed in real time and real space.
Colloids with similar charge will repel one another. This repulsive electrostatic force is lessened, “screened,” by ions present in the medium. However, in a medium with almost no ions, this repulsion can still carry very far, resulting in a long-range repulsion between particles. One such medium is cyclohexylchloride (CHC, dielectric constant ε=7.6) which can easily be deionized to 2-5 pS cm-1.
By incorporating a fluorescent dye in the colloids during particle synthesis, they can be visualized in real space using Confocal Laser Scanning Microscopy (CLSM). From the real space confocal microscopy data, all particle coordinates (positions) can be extracted with standard particle tracking algorithms [1]. From these particle coordinates, the radial distribution function, g(r), can be obtained. The g(r) shows the probability of finding two particles a certain distance, r, apart. The g(r) is one way of obtaining thermodynamics about the system, as it is related to the interparticle potential, w(r). In the limit of extreme dilution, the g(r) is instead only related to the interparticle pair potential, u(r). [2-5]
Due to the long interaction range of long-range repulsive systems, this system is suitable for investigation of the limits of pairwise additivity.
Figure 1: Schematic representation of project steps. A: colloidal particle synthesis, B: confocal microscopy imaging, C: 3D imaging (z-stack)/tomography projections, D: particle tracking, E: determining g(r).
[1] http://www.physics.emory.edu/~weeks/idl/, retrieved February 2013
[2] S. H. Behrens and D. G. Grier, Phys. Rev. E 64, 050401(R) (2001)
[3] D. Gazzillo et al., Phys. Rev. E 74, 051407 (2006)
[4] M. E. Leunissen et al., Nature 437 235–40 (2005)
[5] C. P. Royall et al., J. Phys. Condens. Matter 15, S3581–S3596 (2003)
In situ transmission electron microscopy (TEM)
Traditionally, only materials in a dry state could be investigated with an electron microscope, microscope column is kept at high vacuum. However, differential pumping schemes have allowed study of hydrated specimens and the presence of atmosphere up to a few mbar. Recent advances in microfabrication technology have made it possible to produce nano-compartments that are physically separated from the column vacuum. Strong and electron-transparent membranes^1 separate the sample environment from the column while allowing the radiation through and are aptly termed “windows.” Using two of these windows (separated by a thin spacer) a compartment can be created that allows observation of samples in gaseous environments and even in liquids^2.
Using such a sample cell, we investigate the hydration reaction of MgO in situ:
MgO + H2O -> Mg(OH)2
This type of hydration reaction is important in Earth Sciences^3.
[1] M. J. Williamson et al., Nature Mater. 2, 532–536 (2003)
[2] H. Zheng et al., Science 324, 1309 (2009)
[3] O. Plümper, et al., Geology 40, 1103-1106 (2012)